Everything You Need To Know About Energy Storage Systems
16 Oct, 20199:37Lower costs in renewable technology and a rising global demand for clean energy has seen the...
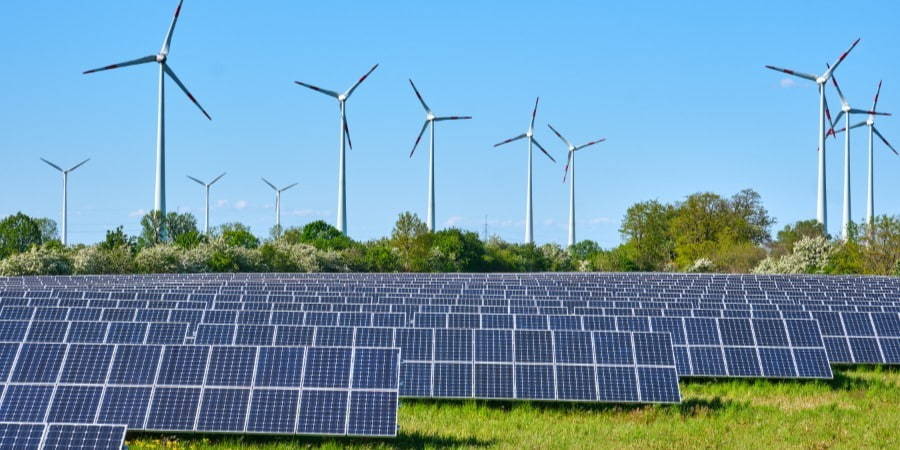
Lower costs in renewable technology and a rising global demand for clean energy has seen the market for wind, solar and tidal energy sources grow exponentially over the last few years, with the trend predicted to continue. According to Allied Market Research, the renewable energy market was valued at $928 billion in 2017, and is expected to reach $1,512.3 billion by 2015.
Due to the inherent limitations of renewable energy sources, the rise in this technology brings with it a growth in a secondary industry - energy storage.
The intermittent nature of a power supply provided by renewable sources such as wind or solar creates a necessity for efficient, scalable ways to store and supply electricity. So when the wind stops blowing or the sun stops shining, grid operators are still able to provide a steady stream of power for uninterrupted use.
As such, the global energy storage market is growing fast. The latest projections see the industry growing by $97.8 billion by 2025.
“Battery energy storage system (BESS) is regarded as a crucial solution for overcoming the intermittency limitations of renewable energy sources,” said Sneha Susan Elias, Senior Analyst of Power at GlobalData.
“The battery energy storage market reported cumulative deployment of 4.9 GW at the end of 2018 and is expected to reach 22.2 GW in 2023 — with the U.S. accounting for 24.7% of the global capacity. The deployment is expected to grow, due to a large number of countries opting for storage utilization to support their power sector transformation.”
So what are the primary technologies being developed that will expand this sector? Read on to find out about all the most effective methods for storing electricity at a large scale.
Batteries
The most common example of energy storage system is the large scale battery. Ongoing innovation in battery technology has continually improved the longevity, capacity and reliability of batteries so that there are now several variations that can be adapted to be suitable for grid-scale developments.
The most widely used form is lithium-ion batteries. First made available for commercial usage in 1991, lithium-ion batteries were initially only developed for consumer electronics, but have been developed by several companies into larger-format cells that can be used in energy storage applications.
The flexibility and the reliability of the technology is a huge benefit that makes these batteries superior in many ways to other forms of rechargeable batteries, and for that reason its developers - Dr. M. Stanley Wittingham, Dr. John Goodenough and Dr. Akira Yoshino - were awarded the 2019 Nobel Prize in Chemistry.
Though it had been previously suggested that Lithium could be used to store energy, it was Dr. Whittingham who first devised a way of doing so at room temperature without the risk of explosion, while working at ExxonMobil.
He and his team found that when lithium ions were held between plates of titanium sulfide they could move back and forth between positive and negative contacts, creating electricity.
Working with Dr. Goodenough at the University of Texas at Austin in 1980, the two scientists were able to improve upon this breakthrough by incorporating a lithium cobalt oxide, which prevented the lithium’s tendency to form needs and dendrites during the recharging process - an unfortunate effect that would cause internal short circuits in the batteries.
Finally, on the other side of the world, Dr. Yoshino took the two scientists’ research and incorporated graphite instead of metallic lithium as the negative electrode - improving the lifespan of the batteries and making them commercially viable for the first time.
Recent developments in lithium-ion battery technology
This was not the end of development of the lithium-ion battery however. Though they were initially only developed for consumer electronics - and are prevalent in rechargeable devices such as mobile phones and laptops - companies continued to develop larger format cells that could be used for large energy storage applications.
The flexibility of the technology has made them essential in the electric vehicle industry, where they can be adapted as small high-power batteries for hybrid power buffering, to medium power batteries that can provide both electric power and buffering in plug-in hybrids, to the increasingly effective high-energy batteries in electric-only vehicles.
And in the last few years they have begun to form the basis of major grid-scale storage developments, the largest of which was built by Tesla to operate with the Hornsdale wind farm in Australia.
The $90 million development was installed in December 2017, having been built in just 100 days. It provides a 100 megawatt emergency electricity backup for up to 30,000 homes. According to the development partner Neoen, the facility saved consumers $40 million within its first year.
There are many benefits to lithium-ion batteries, which is why in a 2016 study it was found that this technology accounted for 95% of deployed systems in the grid-scale battery market. However, there are downsides which are driving demand for other forms of battery.
The downsides of lithium-ion batteries
Since the batteries aren’t themselves designed for industrial use, there is a significant cost in developing them at scale. And while they are extremely cost effective for storage durations of 30 minutes to three hours, they are less efficient when required for longer storage.
They can have robustness issues, with batteries liable to explode if damaged. Over time they will also degrade and require replenishment. Lithium itself is a relatively rare mineral that must be mined at increasing costs, plus there are several sustainability issues with disposal of old batteries.
Alternative batteries
For these reasons there has been an increased push in the development of alternative batteries, with a rise in other forms in grid-scale developments over the last few years.
Redox flow batteries
Redox flow batteries are another class of electrochemical energy storage devices, that was studied by NASA for the space program in the 1970s. The name “redox” refers to the chemical reduction and oxidation reaction that takes place in a liquid electrolyte solution which flows through a battery of electrochemical cells during charge and discharge, storing energy.
Redox flow batteries are extremely adaptable, with the power (kW) being based on the size of the power stack, while the energy capacity (kWh) is a separate factor based on the storage tank size and volume of electrolytes in the tanks. This means that in theory any combination of energy and power can be configured.
Flow batteries can have an extremely long cycle life - lasting tens of thousands of cycles with no inherent degradations. So although they may be more expensive to build than lithium-ion, their cells may not need to be replaced as frequently.
However, they do have a lower energy density than a comparable lithium solution, so to produce the same level of power requires a much larger area. This means they’re not as optimal for electric vehicles - where a low power density can translate as a short driving range (though they can be charged very quickly, so may be useful for hybrids) - but may still be suited to onshore wind or solar farms where space is less of an issue.
Zinc-hybrid batteries
Another battery technology gaining more traction is zinc-hybrid. The first of these rechargeable batteries was used in 1996, to power small and mid-size buses in Singapore. These batteries use a porous anode made from a mass of zinc particles which is then saturated with an electrolyte during discharge.
Hydroxyl ions formed at the cathode by an oxygen reaction move towards the anode to form zincate, which released electrons that travel to the cathode. This is optimal for a four-hour energy discharge, similar to flow batteries.
Though the batteries have a lower efficiency than lithium (approximately 65%-70%) and a similar lifespan that requires replenishment of the cells, zinc is a more widely available and less expensive material than lithium. It’s also easier to source and dispose of ethically and environmentally.
Rechargeable zinc-hybrid battery systems are a relatively new technology for grid-scale storage. In June 2019, Birmingham-based Bryt Energy and Eos Energy Storage announced plans to begin testing the EU’s first zinc hybrid battery system, with plans to roll it out in the UK.
Other companies are also developing larger energy storage solutions using zinc-hybrid batteries to take advantage of the cost benefits. With the technology being at an earlier stage in the commercialisation process, costs have further to fall than other solutions.
Each battery technology has its own benefits and challenges, particularly when scaled up to operate on the grid. Though lithium-ion remains the most popular solution, as development and testing of these and other battery technologies continue, there may be more efficient, reliable and cost-effective options that will form the future of battery energy storage.
Thermal Energy Storage
Thermal energy storage converts energy into heat that can be reused at a later time. The basic process that it undergoes is relatively simple compared to the complex chemical processes that make up batteries, but the solutions at scale can require huge construction projects.
There are three main forms of thermal energy storage systems.
Sensible heat storage
Sensible heat storage is the most commonly used form of thermal energy storage, and the most (relatively) simple to implement. Sensible heat systems use energy to heat or cool solid or liquid matter such as water, salt, sand or rocks.
In solar power systems, for example, the sun’s energy is used to heat up water or salt in an insulated container. The hot water or salt can then be kept for several hours until it is needed, when it can be used to create steam and drive a turbine, turning that energy into electricity.
One of the world’s largest examples of this is the Crescent Dunes Solar Energy Project, based in Nevada. It involves a 10,000 billboard-sized mirrors that reflect sunlight onto a central receiver at the top of a 640 foot tower.
This sunlight heats molten salt directly, to temperatures of 1050F, at which point it is transferred to a hot tank. It can then gradually be fed into a steam generator. In July 2016, the operators ran the plant for a continuous 120-hour period, during which time it delivered 24 hour solar power to the grid.
Latent heat storage
The next form of thermal energy storage is latent heat storage. This less commonly used method relies on the storage medium changing states - eg. solid to liquid. This can be used in applications such as air conditioning or refrigerator technology freezing water at night, then allowing that ice to slowly melt and cool a building during the day - balancing out peak energy demands.
Though latent heat storage is a more expensive approach, it does produce an energy density three times greater than sensible heat storage, meaning the right design in a grid scale system may prove beneficial.
Thermo-chemical storage
The final form of thermal energy storage is thermo-chemical storage, which uses chemical reactions to store energy. This approach is considered the most energy efficient, with thermochemical reactions yielding the highest storage capacity.
Chemicals used include silica gel/water, magnesium sulfate/water, lithium bromide/water, lithium chloride/water, and NaOH/water. The process involves using heat to evaporate water and create an endothermic reaction. When water is added again it creates an exothermic reaction, which generates heat that can be used to generate electricity.
Since salt chemicals formed by the initial reaction can be kept for long periods of months or even years, this approach can be advantageous for seasonal energy storage - eg. using solar power from summer to generate heat in winter.
However, the equipment and materials required for any thermo-chemical reactor is significantly more expensive than other solutions. There is also an issue with effectively sealing the chemicals at scale.
Currently most thermal energy storage systems use a sensible heat process, though significant research and development activity is being put into latent heat and thermo-chemical heat storage, which could result in greater future usage.
Mechanical Energy Storage
Mechanical energy storage systems use kinetic or gravitational forces to store energy. Since generators use the movement of a turbine to generate electricity, these systems harness the potential force to drive that turbine for a later date.
Like thermal energy storage, it’s based off a relatively simple theory, but produces some complex and imaginative results. In its simplest form it can take the shape of a weight and pulley, with the energy required to lift the weight stored as gravitational potential until it is released again. But more ambitious ideas are required in order to store grid-scale energy.
Pumped storage hydroelectricity is one major form that has been in use since the 1980s. The largest pumped storage station currently is Bath County in Virginia. Like all hydroelectric dams it uses the force of rushing water to turn a turbine and generate power, with the dam controlling the flow of water to optimise generation.
Where this differs from standard hydroelectric dams, is that it “stores” energy by using power during times of low demand to pump water from the lower to the upper reservoir. When demand is higher, this water can then be released again through the turbine.
Another concept that utilises gravitational potential to store energy is a design by Swiss company Energy Vault, that recently received major investment of $110 million from a Japanese holding company Softbank. Their concept is a tower of concrete blocks that can be lifted by a series of cranes.
The company intends to use these blocks alongside wind farms, with excess power from the wind turbines used to operate the cranes. When demand is higher, the concrete blocks are lowered back to the ground. The kinetic energy generated as they fall will generate electricity, unleashing the stored energy. According to the designers, these blocks have an energy efficiency of around 90%.
Hydrogen (power to gas) energy storage
Energy can be stored through the electrolysis of water, which separates hydrogen and oxygen molecules. Hydrogen can then be stored and then used to generate electricity in much the same way as fossil fuels - but without any emissions. It’s also used effectively in fuel cells for the automotive sector.
When used in a small scale, hydrogen can be stored in pressurised vessels. But for large scale projects it is stored in underground salt caverns of up to 500,000 cubic metres, at pressures of 2,900 psi. A cavern at this size and pressure would deliver a capacity of around 100 GWh of stored electricity.
According to a market research report released in October 2019 by MarketsandMarkets, the global hydrogen energy storage market is currently $13.7 billion, and projected to reach $18.2 billion by 2024.
Using this for grid scale projects is a relatively new development. Largescale projects include Germany’s Energiepark Mainz - a 6MW electrolysis and storage project - and the USA’s Markham Energy Storage Facility - a 2.5MW facility in Ontario that has been designed and built on a 5MW scalable platform.
Efficiency is a problem for power to gas systems, with round-trip efficiency averaging less than 50% in 2013. However, new research has suggested ways of improving this, with a 2019 study suggesting that up to 80% may be feasible using pressurised reversible solid oxide fuel cells.
As renewable energy grows more prevalent, so does the need for efficient, scalable, cost-effective energy storage. However it remains a challenge to find an optimal solution that will fit all situations. It’s likely that further development on each method of energy storage will continue, with widely varying facilities being developed alongside renewable energy projects throughout the world.
Work on the future of energy with NES Fircroft
NES Fircroft has been working with some of the world’s biggest energy companies for over 50 years. Our renewable energy recruitment teams possess extensive experience recruiting technical and commercial professionals across multiple international locations.
Register with NES Fircroft for free to receive the latest updates on available jobs for engineering and technical professionals.